Hello, ๐
The business landscape is in a state of constant transformation, with sustainability taking center stage in recent years. Numerous innovative sectors have emerged, including renewable energy, green finance, and the circular economy. Each technological and environmental advancement promises to reshape industries, yet the fundamental structure of the market endures, accommodating these new domains within its fold.
The latest game-changer is the carbon business, encompassing carbon trading, carbon offsetting, and carbon capture. Its core value lies in mitigating climate change by reducing greenhouse gas emissions and promoting sustainable practices. In this article, we will delve into Carbon Business Ecosystem: Trends, Challenges, and Opportunities. Happy reading!
Garvit Sahdev enjoys understanding ideas that shape our world. The Thoughtful Tangle is an initiative to share this journey and experience with his friends who love to do the same. He selects one idea and dives deep into it to understand its basics, relevance, impact and opportunities around it. The thoughtful tangle is special because ๐
๐ One long article per idea. We call it โThe Basicsโ.
๐ Multiple unique insights. Separate small articles for special โInsightsโ.
๐งโ๐คโ๐ง Experts perspective. Check out โInsidersโ
What is Climate Change?
Climate change refers to significant and lasting changes in the Earth's climate patterns over an extended period. It encompasses both global warming, driven primarily by human activities, and broader changes in weather patterns. The primary cause of recent climate change is the increase in greenhouse gases (GHGs) in the atmosphere, which enhances the greenhouse effect and leads to global warming.
Climate Model
A climate model can be built to understand the relationship between various factors affecting the Earth's climate.
The model assumes the Earth is a single point that absorbs sunlight and emits infrared radiation.
Insolation (Incoming Solar Radiation): The amount of solar energy received by the Earth.
where S is the solar constant (~1361 W/mยฒ), L is the solar luminosity, and A is the albedo (reflectivity) of the Earth (~0.3).
Outgoing Longwave Radiation (OLR): The Earth's surface emits energy as blackbody radiation.
where โsigmaโ is the Stefan-Boltzmann constant (5.67 ร 10โปโธ W/mยฒKโด) and T is the Earth's temperature in Kelvin.
At equilibrium, the incoming solar radiation equals the outgoing longwave radiation.
The greenhouse effect can be incorporated by considering the additional warming due to greenhouse gases, which trap some of the outgoing infrared radiation.
The Greenhouse Effect
The greenhouse effect is the process by which greenhouse gases (GHGs) in the Earth's atmosphere trap heat. This mechanism is critical for maintaining the planet's temperature at a level suitable for life. Hereโs how it works:
The Earth absorbs solar radiation and warms up.
The Earth emits infrared radiation (heat) back towards space.
GHGs like carbon dioxide (COโ), methane (CHโ), water vapor (HโO), and nitrous oxide (NโO) absorb some of this infrared radiation and re-radiate it in all directions, including back towards the Earth's surface.
This process reduces the amount of heat escaping to space, effectively insulating the planet and causing additional warming of the surface and lower atmosphere.
Radiative/Climate Forcing
Radiative forcing is the change in energy flux in the atmosphere due to factors such as greenhouse gases, aerosols, and solar radiation. It is measured in watts per square meter (W/mยฒ).
Positive Forcing:
Increases energy in the Earth system, leading to warming. Examples include increased GHG concentrations and black carbon (soot).
Negative Forcing:
Decreases energy in the Earth system, leading to cooling. Examples include aerosols like sulfate particles that reflect sunlight.
Calculating Radiative Forcing
For example, the radiative forcing of COโ can be calculated using:
where โDelta Fโ is the radiative forcing, C is the current concentration of COโ, and Cโ0โ is the pre-industrial concentration.
The Drivers of Climate Change
Climate change is driven by various natural and anthropogenic factors:
Natural Drivers:
Solar Variability: Changes in solar radiation due to solar cycles.
Volcanic Activity: Eruptions can inject aerosols into the atmosphere, reflecting sunlight and causing short-term cooling.
Anthropogenic Drivers:
Greenhouse Gas Emissions: Burning fossil fuels, deforestation, and industrial activities increase the concentration of GHGs.
Land Use Changes: Urbanization and deforestation alter the Earth's albedo and carbon cycle.
Aerosols: Industrial activities release aerosols that can have cooling or warming effects depending on their properties.
Climate Science
Climate science encompasses a broad range of research activities aimed at understanding the Earth's climate system, predicting future climate changes, and assessing their impacts. Here's an in-depth look at various aspects of climate science:
Earth's Temperature Observations:
Historical Records:
Temperature records date back to the 19th century and include measurements from land-based weather stations, ships, and buoys.
Historical temperature data show a long-term warming trend, with significant increases since the mid-20th century.
Climate Sensitivity
Climate sensitivity refers to the equilibrium temperature change in response to a doubling of atmospheric CO2 concentration relative to pre-industrial levels.
Key Metrics:
1. Equilibrium Climate Sensitivity (ECS):
ECS estimates the long-term change in global mean surface temperature after CO2 doubling, including slow feedback like ice sheet responses. Typical estimates range from 1.5ยฐC to 4.5ยฐC.
2. Transient Climate Response (TCR):
TCR measures the temperature change at the time of CO2 doubling, assuming a gradual increase in CO2 (1% per year), focusing on more immediate feedback and excluding slower processes.
How Climate Models Work
Climate Models:
Climate models simulate the interactions of the atmosphere, oceans, land surface, and ice. They are based on physical principles and are used to predict future climate changes.
Types of Models:
1. General Circulation Models (GCMs):
These complex models represent the Earthโs climate system using three-dimensional grids, solving equations for atmospheric and oceanic flows, energy exchanges, and biogeochemical cycles.
2. Earth System Models (ESMs):
ESMs extend GCMs by including interactions between the climate and biological processes, such as carbon cycling and vegetation dynamics.
3. Regional Climate Models (RCMs):
RCMs provide higher resolution climate projections for specific regions by downscaling results from GCMs.
Modeling Process:
1. Initialization:
Models are initialized with current and historical data, including temperature, CO2 levels, and other climatic variables.
2. Simulation:
Models simulate climate processes over time, often using scenarios that represent different future pathways of greenhouse gas emissions (e.g., RCPs or SSPs).
3. Validation:
Model outputs are validated against observed data to ensure accuracy and reliability.
Organizations Doing Climate Science
1. Intergovernmental Panel on Climate Change (IPCC):
The IPCC assesses scientific knowledge on climate change, providing comprehensive reports that inform global policy.
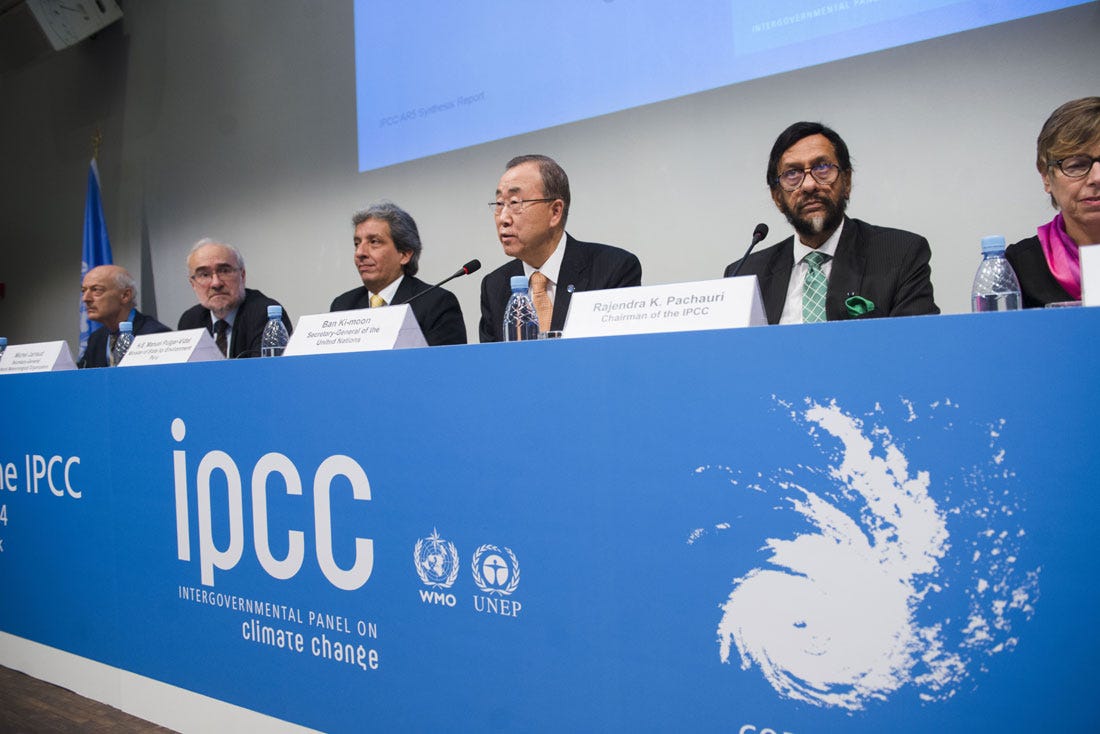
2. National Aeronautics and Space Administration (NASA):
NASA conducts climate research using satellite data, climate modeling, and field experiments to understand climate dynamics and trends.
3. National Oceanic and Atmospheric Administration (NOAA):
NOAA monitors and studies climate, weather, oceans, and coasts, providing valuable climate data and projections.
4. World Meteorological Organization (WMO):
The WMO facilitates international cooperation in climate science and monitoring, standardizing data collection and sharing.
5. Climate Research Units and Universities:
Institutions like the Hadley Centre for Climate Science and Services (UK), Scripps Institution of Oceanography (USA), and others conduct cutting-edge research and contribute to global climate knowledge.
Emissions and the Global Carbon Cycle
The Global Carbon Cycle
The global carbon cycle describes the movement of carbon among various reservoirs on Earth, including the atmosphere, oceans, terrestrial biosphere, and lithosphere. Understanding this cycle is essential for grasping how human activities impact climate change.
Key Components of the Carbon Cycle:
1. Atmospheric Carbon:
Carbon dioxide (COโ) and methane (CHโ) are the primary carbon-containing gases in the atmosphere. They play a significant role in the greenhouse effect.
2. Terrestrial Biosphere:
Forests, soil, and plants absorb COโ through photosynthesis and store carbon in biomass and soil organic matter. Respiration by plants and decomposition of organic matter release COโ back into the atmosphere.
3. Oceans:
Oceans absorb COโ from the atmosphere. Carbon is stored in dissolved forms such as bicarbonate and carbonate ions. Marine organisms also sequester carbon in their shells and skeletons, which can become part of ocean sediments.
4. Lithosphere:
The largest carbon reservoir, storing carbon in rocks, fossil fuels, and sediments. Weathering of rocks, volcanic activity, and the formation and burning of fossil fuels influence the carbon cycle.
Processes in the Carbon Cycle:
1. Photosynthesis and Respiration:
Plants convert COโ and sunlight into organic matter and oxygen. Respiration by plants, animals, and microbes returns COโ to the atmosphere.
2. Ocean-Atmosphere Exchange:
COโ dissolves in seawater and is exchanged between the ocean and atmosphere. This process is influenced by temperature, ocean currents, and biological activity.
3. Fossil Fuel Combustion:
Burning fossil fuels releases significant amounts of COโ and CHโ, altering the natural carbon balance.
4. Deforestation and Land Use Changes:
Deforestation reduces the capacity of the terrestrial biosphere to absorb COโ, while land-use changes can release stored carbon from soils and vegetation.
Global Warming Potential
Global Warming Potential (GWP) is a measure of how much heat a greenhouse gas traps in the atmosphere over a specific time period, compared to COโ. It helps compare the impacts of different gases on global warming.
Key Points:
1. Reference Gas:
COโ is the baseline with a GWP of 1 over a 100-year period.
2. Methane (CHโ):
Methane has a GWP of about 28-36 over 100 years. Despite its shorter atmospheric lifetime (~12 years), it is much more effective at trapping heat compared to COโ.
Sources: Natural sources include wetlands and termites. Anthropogenic sources include livestock digestion, rice paddies, landfills, and fossil fuel extraction.
Impact: Methane is a powerful greenhouse gas with a short atmospheric lifetime but significant immediate impact on warming.
3. Nitrous Oxide (NโO):
Nitrous oxide has a GWP of about 298 over 100 years and a longer atmospheric lifetime (~114 years).
Emitted from agricultural activities, industrial processes, and combustion of organic matter and fossil fuels.
4. Fluorinated Gases:
Synthetic gases like hydrofluorocarbons (HFCs) can have GWPs ranging from hundreds to tens of thousands, making them potent climate forces.
Includes HFCs, perfluorocarbons (PFCs), and sulfur hexafluoride (SFโ). They are used in industrial applications and as refrigerants.
5. Water Vapor (HโO):
The most abundant greenhouse gas acts as feedback rather than a direct force because its concentration in the atmosphere is controlled by temperature.
Emissions Accounting
Emissions accounting involves tracking and reporting greenhouse gas emissions to understand and manage their impact on climate change. It is crucial for setting and meeting targets for emission reductions.
Key Elements:
1. Scope:
Scope 1: Direct emissions from owned or controlled sources.
Scope 2: Indirect emissions from the generation of purchased electricity, steam, heating, and cooling.
Scope 3: All other indirect emissions in the value chain, including those from the production of purchased goods and services, transportation, and waste.
2. Inventories:
National and organizational greenhouse gas inventories quantify emissions from various sectors, including energy, industrial processes, agriculture, and waste.
3. Reporting Standards:
Protocols like the Greenhouse Gas Protocol and standards from the International Organization for Standardization (ISO) provide guidelines for accurate and consistent emissions reporting.
4. Carbon Foot printing:
Calculating the total greenhouse gas emissions caused directly and indirectly by an individual, organization, event, or product.
5. Verification:
Independent verification ensures the accuracy and credibility of emissions data and reports.
Understanding Global Impacts
1.5ยฐC Target:
The 1.5ยฐC target is a threshold identified by climate scientists and adopted by the Paris Agreement to limit global warming to 1.5ยฐC above pre-industrial levels.
Importance:
Avoiding Severe Impacts: Limiting warming to 1.5ยฐC can significantly reduce the risks of extreme weather events, loss of biodiversity, and disruption to ecosystems and human systems.
Mitigating Risks: Beyond 1.5ยฐC, the likelihood of triggering tipping points and irreversible changes increases, including the collapse of major ice sheets and substantial sea-level rise.
Ceasing Global Warming:
Emissions and Temperature: Global warming will stabilize only when human-caused emissions of greenhouse gases (GHGs) reach net zero. This means any remaining emissions are balanced by removal from the atmosphere.
Time Lag: Even after achieving net-zero emissions, some warming will persist due to the long-lived nature of COโ and other GHGs already in the atmosphere.
Climate Inertia: The climate system has inertia, meaning oceans and ice sheets will continue to respond to past emissions, leading to ongoing changes for decades to centuries.
Global Impacts
Temperature:
Increase: Global temperatures have risen approximately 1.1ยฐC above pre-industrial levels and are projected to continue increasing if emissions are not curbed.
Heatwaves: More frequent and severe heatwaves are expected, impacting human health, agriculture, and natural ecosystems.
Rainfall:
Patterns: Changes in precipitation patterns, with some regions experiencing more intense rainfall and others facing increased droughts.
Extreme Events: Increased frequency and intensity of extreme weather events like storms and hurricanes.
Ice and Snow:
Melting: Accelerated melting of glaciers, ice caps, and polar ice sheets contributes to rising sea levels.
Permafrost Thawing: Thawing permafrost releases methane, a potent greenhouse gas, creating a feedback loop that accelerates warming.
Sea Level:
Rise: Sea levels have risen due to thermal expansion of seawater and melting ice, and are projected to rise further, threatening coastal communities and ecosystems.
Flooding: Increased risk of coastal flooding, erosion, and saltwater intrusion into freshwater sources.
Ocean Currents:
Disruption: Changes in temperature and salinity can alter major ocean currents, affecting global climate patterns and marine ecosystems.
Examples: The slowdown of the Atlantic Meridional Overturning Circulation (AMOC) could have significant impacts on regional climates.
Global Carbon Budget
The global carbon budget refers to the maximum amount of COโ that can be emitted while still having a likely chance of limiting global warming to a specific temperature target, such as 1.5ยฐC or 2ยฐC.
Current Estimates:
For a 67% chance of limiting warming to 1.5ยฐC, the remaining carbon budget is around 400-500 gigatons of COโ (GtCOโ).
For a 2ยฐC target, the budget is larger but still finite, emphasizing the urgency of reducing emissions.
Making Sense of Emissions Reduction Scenarios
Emissions reduction scenarios, such as those developed by the Intergovernmental Panel on Climate Change (IPCC), outline pathways to achieve climate targets. These scenarios vary based on the speed and extent of emissions reductions and the deployment of carbon removal technologies.
Key Approaches:
Immediate Reductions: Drastic and immediate reductions in GHG emissions are crucial to stay within the carbon budget.
Technological Solutions: Adoption of renewable energy, electrification of transport, and improvements in energy efficiency.
Negative Emissions: Techniques such as afforestation, reforestation, and direct air capture to remove COโ from the atmosphere.
Tipping Points:
Critical thresholds where a small change can lead to drastic and irreversible shifts in the climate system.
Examples:
Melting of the Greenland and West Antarctic ice sheets.
Collapse of the Amazon rainforest due to deforestation and climate change.
Carbon Cycle Feedbacks:
Positive Feedbacks: Processes that amplify initial warming. Examples include:
Permafrost Thawing: Releases methane and COโ, enhancing warming.
Ice-Albedo Feedback: Melting ice reduces surface reflectivity, causing more heat absorption and further melting.
Negative Feedbacks: Processes that mitigate warming. Examples include increased plant growth due to higher COโ levels, though these are generally less impactful than positive feedbacks.
Science of Extreme Event Attribution
Extreme event attribution is a field of climate science that investigates the influence of human-induced climate change on the frequency and intensity of extreme weather events such as heatwaves, droughts, floods, and storms.
Methodology:
Statistical Analysis: Comparing observational data of extreme events with historical climate data to identify trends and anomalies.
Climate Models: Using climate models to simulate conditions with and without human influences to estimate the probability of extreme events occurring in each scenario.
Event Probability: Quantifying how climate change has altered the likelihood or severity of specific extreme events. This involves calculating the Fraction of Attributable Risk (FAR):
Key Findings:
Many studies have found that climate change has increased the probability and intensity of heatwaves, heavy precipitation events, and some types of storms.
What is a Disaster?
A disaster is a serious disruption of the functioning of a community or society causing widespread human, material, economic, or environmental losses that exceed the community's ability to cope using its own resources.
Types of Disasters:
1. Natural Disasters: Includes extreme weather events (e.g., hurricanes, floods), geological events (e.g., earthquakes, tsunamis), and biological events (e.g., pandemics).
2. Human-Induced Disasters: Includes industrial accidents, environmental pollution, and climate change-induced events.
Disaster Impact Factors:
Exposure: The presence of people, livelihoods, and assets in areas that could be adversely affected by hazards.
Vulnerability: The susceptibility of a community to harm due to social, economic, and environmental factors.
Capacity: The ability of a community to anticipate, cope with, and recover from the impacts of disasters.
Cumulative Impacts
The aggregate effect of multiple extreme events and long-term climate changes over time, which can exacerbate vulnerabilities and reduce resilience.
Examples:
1. Agricultural Impacts: Repeated droughts and heatwaves can lead to crop failures, soil degradation, and food insecurity.
2. Economic Impacts: Accumulated damage from frequent storms and floods can strain economic resources, increase insurance costs, and disrupt livelihoods.
3. Health Impacts: Long-term exposure to air pollution, heatwaves, and the spread of vector-borne diseases can have severe public health consequences.
4. Ecological Impacts: Persistent changes in temperature and precipitation patterns can lead to habitat loss, biodiversity decline, and changes in ecosystem services.
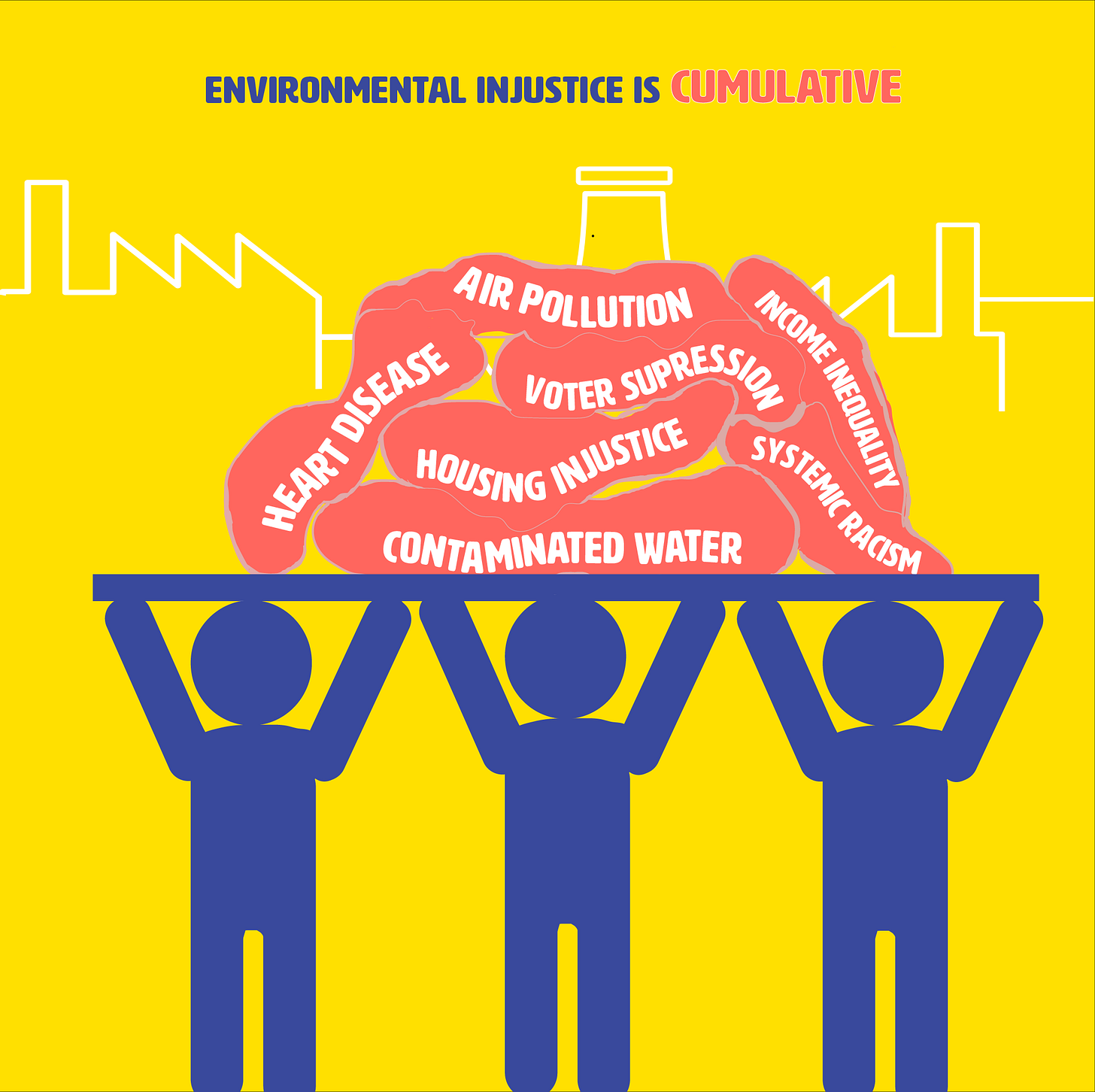
Communicating Impacts Through Stories
Personal stories and local narratives can make abstract scientific concepts more relatable and compelling, fostering greater public understanding and engagement.
Effective Storytelling Strategies:
Human Interest Stories: Highlighting personal experiences and testimonials of those directly affected by climate change to create emotional connections.
Localized Context: Using familiar settings and community-specific examples to illustrate the impacts of climate change.
Visual Aids: Incorporating photographs, videos, and infographics to visually communicate the effects of extreme events and cumulative impacts.
Solutions-Oriented: Balancing stories of impacts with narratives about adaptation and mitigation efforts to inspire action and hope.
Solutions to Climate Change
Evaluating and comparing different approaches to tackling the climate crisis involves considering various perspectives and criteria. Hereโs a detailed exploration of the key concepts and lenses that can be used for such evaluations:
Mental Models
Mental models are frameworks or thought processes that individuals use to understand and interpret the world.
Application in Climate Solutions:
- Systems Thinking: Viewing the climate as a complex system with interconnected components. Solutions must consider feedback loops, delays, and interdependencies.
- Risk Management: Focusing on identifying, assessing, and prioritizing risks, then applying resources to minimize and manage those risks.
- Economic Models: Using cost-benefit analysis, discount rates, and market-based solutions like carbon pricing to evaluate climate strategies.
Evaluating Approaches:
- Holistic Understanding: Effective climate solutions require integrating multiple mental models to address the complexity of the climate system and the socio-economic impacts.
Comparing Impact and Cost
Impact:
- Assessing the effectiveness of a solution in reducing greenhouse gas emissions, enhancing resilience, and achieving long-term sustainability goals.
- Metrics: Carbon reduction potential, adaptation capacity, biodiversity preservation, and social benefits.
Cost:
- Evaluating the financial requirements of implementing solutions, including initial investment, operation and maintenance costs, and potential economic benefits or savings.
- Metrics: Cost per ton of COโe reduced, return on investment (ROI), and cost-effectiveness.
Comparison Frameworks:
- Marginal Abatement Cost Curve (MACC): A tool to compare the cost-effectiveness of various emissions reduction measures.
- Cost-Benefit Analysis (CBA): Comparing the costs and benefits of different interventions to determine their overall value.
Masculine vs. Feminine Framings
Masculine Framing:
- Often focuses on technology-driven, large-scale solutions, competition, and control over nature.
- Examples include geoengineering, large-scale renewable energy projects, and technological innovations.
Feminine Framing:
- Emphasizes community-based, nurturing, and cooperative approaches, and living in harmony with nature.
- Examples include grassroots movements, regenerative agriculture, and decentralized renewable energy systems.
Evaluating Framings:
- Inclusivity: Effective climate action requires integrating both masculine and feminine framings to harness technological advancements while fostering community engagement and equity.
Growth vs. Degrowth
Growth:
- Pursues sustainable economic growth through green technologies, renewable energy, and efficiency improvements.
- Pros: Potential for innovation, job creation, and maintaining or enhancing living standards.
- Cons: May still lead to resource overuse and environmental degradation if not managed properly.
Degrowth:
- Advocates for reducing consumption, economic contraction, and prioritizing ecological and social well-being over GDP growth.
- Pros: Potential to reduce environmental impact and promote equitable resource distribution.
- Cons: Challenges include potential economic instability and resistance from growth-dependent societies.
Evaluating Approaches:
- Sustainability Metrics: Assessing whether growth or degrowth strategies lead to sustainable resource use and long-term environmental health.
- Equity and Well-Being: Evaluating the impact on social equity, quality of life, and resilience.
Systems and Justice Lenses
Systems Lens:
- Analyzing climate solutions within the context of larger systems, recognizing interconnections and potential unintended consequences.
- Examples: Considering the food-water-energy nexus, urban-rural linkages, and global supply chains.
Justice Lens:
- Prioritizing equity, fairness, and justice in climate action, ensuring that vulnerable and marginalized communities are not disproportionately affected.
- Examples: Climate justice, environmental justice, and ensuring just transitions for workers in fossil fuel industries.
Evaluating Approaches:
- Intersectionality: Assessing how solutions impact different social groups and addressing systemic inequalities.
- Long-Term Viability: Ensuring solutions are resilient and sustainable, benefiting both current and future generations.
Communicating Climate Change: Key Strategies
Effectively communicating climate change requires understanding your audience and tailoring your message accordingly. Here's a breakdown of the key points you mentioned:
1. Identifying Shared Values:
Find common ground: Climate change can be a polarizing topic. Focus on values everyone shares, like protecting our planet, ensuring a healthy future for generations to come, or fostering economic stability.
Frame the message around these values: Highlight how climate change threatens these values and how solutions can safeguard them. For example, talk about climate-resilient agriculture to ensure food security or green jobs to boost the economy.
2. Dismissives, Deniers, and Doubters:
Acknowledge their concerns: Don't dismiss opposing viewpoints. Listen to their doubts and address them with credible information.
Use data and stories: Facts and figures are important, but statistics can be overwhelming. Use clear, concise data and relatable stories to illustrate the impact of climate change.
Appeal to their sense of logic: Present evidence in a way that is easy to understand and follow.
3. Centering Solutions:
Focus on hope and action: Climate change can be scary. Shift the conversation towards solutions and what individuals and communities can do to create positive change.
Empower your audience: People are more likely to take action if they feel they can make a difference. Provide concrete steps individuals can take in their daily lives.
Showcase successful initiatives: Highlight existing solutions like renewable energy projects or sustainable practices that are making a positive impact.
4. Corporate and Media Contexts:
Tailor your message to the audience: When talking to corporations, emphasize the economic benefits of sustainability and climate action. For media audiences, focus on newsworthy angles and impactful visuals.
Find credible sources: Back your claims with data from reputable scientific institutions and organizations.
Be mindful of media bias: Be aware of potential bias in media outlets and frame your message accordingly.
By understanding your audience and using these strategies, you can communicate climate change more effectively, leading to increased awareness, action, and ultimately, solutions.
Exploring Creative Solutions
Climate change poses a serious threat to our planet and its inhabitants. Fortunately, innovative models are emerging that promote sustainable development, addressing climate change while improving human well-being. Here's a closer look at some of the models you mentioned:
1. Sarvodaya Shramadana Movement (Sri Lanka):
This Sri Lankan grassroots movement focuses on community-driven development.
Key principles include:
Shramadana: This Sinhalese term translates to "sharing of labor." Villagers contribute their time and skills to work on infrastructure projects, fostering self-reliance and community ownership.
Microfinance: Sarvodaya provides financial resources to local entrepreneurs, empowering communities to develop sustainable livelihoods.
Holistic Development: The movement addresses not just economic needs but also social, educational, and spiritual well-being.
By empowering communities and promoting self-sufficiency, Sarvodaya demonstrates how development can be achieved with minimal environmental impact.
2. Green New Deal (U.S.):
The Green New Deal is a proposed set of policies aiming to address climate change and economic inequality simultaneously. Key goals include:
Transition to renewable energy: Investing in clean energy sources like solar and wind power would reduce dependence on fossil fuels and greenhouse gas emissions.
Infrastructure development: Modernizing infrastructure like the electricity grid is crucial for integrating renewable energy sources effectively.
Job creation: The Green New Deal proposes creating millions of jobs in clean energy sectors, fostering economic growth alongside environmental benefits.
While the Green New Deal remains a proposal, it offers a framework for large-scale economic and environmental transformation.
3. Sustainable Development in Costa Rica:
Costa Rica is a global leader in sustainable development. Here are some key aspects of their approach:
Forestry: Costa Rica has successfully reversed deforestation trends and increased forest cover through ecotourism and payments for ecosystem services.
Renewable Energy: The country relies heavily on renewable energy sources like geothermal and hydropower, making it a model for clean energy transition.
Biodiversity Conservation: Costa Rica has established a vast network of protected areas, preserved its rich biodiversity and promoted sustainable tourism.
Costa Rica's success story demonstrates the positive economic and environmental outcomes achievable through strong policy and public commitment.
4. Doughnut Economics:
The Doughnut Economics model, proposed by Kate Raworth, provides a framework for achieving social justice and environmental sustainability.
The Social Doughnut: Represents the minimum amount of resources needed for everyone to live a decent life (food, healthcare, education, etc.).
The Ecological Doughnut: Represents the ecological boundaries of our planet, beyond which our activities become unsustainable.
The ideal economic system operates within the "doughnut hole," ensuring everyone's needs are met without exceeding ecological limits. This model promotes economic policies that focus on human well-being and environmental sustainability.
These models show a range of approaches to sustainable development. From community-driven initiatives to national policies and economic frameworks, there's a growing toolbox available to navigate the challenges of climate change. By learning from and replicating these successful models, we can pave the way for a more sustainable future.
Economics of Climate Change
Climate Change as Market Failure
Climate change is considered a market failure for several reasons:
Negative Externalities: An externality occurs when a third party is affected by an economic transaction without being involved in it. Greenhouse gas emissions from fossil fuel combustion result in environmental and health damages that are not reflected in the price of the emitting activity. As a result, markets fail to allocate resources efficiently, leading to higher-than-optimal levels of emissions.
Public Goods and the Tragedy of the Commons: The atmosphere, as a public good, is subject to overuse because no one owns it, and everyone can emit into it without directly paying the cost. This leads to the "tragedy of the commons," where individual actors, acting in their self-interest, deplete a shared resource, resulting in overall harm.
Information Asymmetry: There is often a lack of complete information about the environmental impacts of goods and services. Consumers and producers may not fully understand or value the long-term consequences of their actions, leading to decisions that do not account for environmental costs.
Intergenerational Equity: Climate change presents a unique challenge where the costs and benefits of actions are distributed across different generations. Current generations may be less motivated to reduce emissions since the benefits of such actions will primarily be realized by future generations, creating a misalignment in incentives.
Alternatives to Mainstream Environmental Economics
Alternative approaches offer broader perspectives on addressing climate change:
Ecological Economics: This field integrates ecological and economic principles, emphasizing sustainability and the health of ecosystems. It argues for a steady-state economy that prioritizes ecological balance over continuous economic growth. Policies advocated include strong regulation of resource use, protection of natural habitats, and valuing ecosystem services.
Degrowth Movement: Degrowth proponents argue that sustainable well-being cannot be achieved through perpetual economic growth. Instead, they advocate for reducing consumption and production, focusing on societal well-being, and achieving ecological sustainability. Policy measures might include reducing work hours, encouraging local economies, and promoting non-material wealth.
Environmental Justice: This approach emphasizes fair treatment and meaningful involvement of all people regardless of race, color, national origin, or income regarding environmental laws, regulations, and policies. It seeks to address the disproportionate environmental burdens borne by marginalized communities and ensure inclusive and equitable decision-making processes.
Policy Options for Climate Change
Policy options to tackle climate change span various approaches:
Regulatory Measures: These include laws and regulations that directly limit emissions or require certain behaviors. Examples are emission standards for vehicles, renewable energy mandates requiring a certain percentage of energy to come from renewable sources, and building codes that require energy efficiency.
Market-Based Instruments: These tools leverage market mechanisms to encourage emission reductions cost-effectively. Carbon pricing (taxes and cap-and-trade systems) is a prime example, as it internalizes the cost of carbon emissions, incentivizing businesses and consumers to reduce their carbon footprints.
Subsidies and Incentives: Governments can provide financial incentives for activities that reduce emissions, such as subsidies for renewable energy projects, tax credits for energy-efficient appliances, and grants for research and development in green technologies.
International Agreements: Climate change is a global problem requiring coordinated international action. Agreements like the Paris Agreement set emission reduction targets for countries and establish frameworks for monitoring, reporting, and supporting climate action globally.
Principles of Carbon Pricing
Carbon pricing aims to internalize the external costs of carbon emissions:
Carbon Tax: This is a straightforward method where a tax is levied on the carbon content of fossil fuels. It provides a predictable price on carbon, encouraging emitters to reduce their carbon footprint. The tax rate can be adjusted over time to meet emission reduction goals.
Cap-and-Trade System: This system sets a cap on total emissions and allows entities to buy and sell emission allowances. The cap ensures that emission reductions are achieved, while trading allows for flexibility and cost-effectiveness. Entities that can reduce emissions at lower costs can sell their excess allowances to those facing higher costs, leading to overall economic efficiency.
Revenue Recycling: The revenue generated from carbon pricing can be used in various ways, such as reducing other taxes (like income or corporate taxes), investing in renewable energy projects, or providing rebates to low-income households to offset higher energy costs.
Carbon Markets
Carbon markets are mechanisms to trade carbon credits or allowances:
Compliance Markets: These markets are created by regulatory frameworks that require certain sectors to cap their emissions and trade allowances to meet their limits. The European Union Emissions Trading System (EU ETS) is the largest compliance market, covering power plants, industrial facilities, and airlines.
Voluntary Markets: These markets operate outside regulatory frameworks, allowing companies and individuals to voluntarily purchase carbon offsets. Projects that generate these offsets include reforestation, renewable energy installations, and methane capture. While less regulated, voluntary markets can drive additional emission reductions and raise awareness about carbon footprints.
Social Cost of Carbon
The social cost of carbon (SCC) is a crucial metric in climate policy:
Calculation: The SCC is estimated using complex models that project the impacts of carbon emissions on agriculture, health, property damages from sea-level rise, and changes in ecosystem services. These models incorporate discount rates to account for the time value of money and uncertainties in future climate impacts.
Policy Implications: The SCC is used to evaluate the benefits of emission reduction policies and justify regulatory measures. By assigning a monetary value to the damage caused by each ton of CO2 emitted, policymakers can assess the economic efficiency of various climate actions.
Challenges: Estimating the SCC involves significant uncertainties due to the long-term nature of climate impacts, the difficulty in predicting technological advancements, and the complexity of modeling economic and environmental interactions. Different assumptions about discount rates and climate sensitivity can lead to widely varying estimates.
Global Climate Politics and Justice
Global Negotiations and the Paris Agreement
Global Negotiations: Climate change negotiations have been conducted under the United Nations Framework Convention on Climate Change (UNFCCC) since 1992. Key milestones include the Kyoto Protocol (1997), which set binding emission reduction targets for developed countries, and the Copenhagen Accord (2009), which recognized the need to limit global temperature rise to below 2ยฐC but failed to produce a binding agreement.
Paris Agreement: Adopted in 2015, the Paris Agreement is a landmark international accord within the UNFCCC framework. It aims to limit global warming to well below 2ยฐC above pre-industrial levels, with efforts to limit the increase to 1.5ยฐC. Unlike the Kyoto Protocol, the Paris Agreement includes commitments from all countries, both developed and developing, to reduce emissions (Nationally Determined Contributions or NDCs). It also established mechanisms for transparency, reporting, and review, and created the Green Climate Fund to support developing countries in adaptation and mitigation efforts.
Politics, Policies, and Justice
Politics: Climate politics involves complex interactions between international, national, and local levels. Different countries have varying interests based on their economic structures, levels of development, and vulnerability to climate impacts. Political will and leadership, lobbying by fossil fuel industries, and public opinion all play significant roles in shaping climate policy.
Policies: Policies to address climate change include regulatory measures (e.g., emission standards), economic instruments (e.g., carbon pricing), and support for innovation (e.g., subsidies for renewable energy). Effective policies often require balancing environmental goals with economic and social considerations, ensuring that transitions are just and equitable.
Justice: Climate justice emphasizes fair treatment of all people and equitable distribution of the benefits and burdens of climate policies. It involves addressing the disproportionate impact of climate change on vulnerable populations, including low-income communities, indigenous peoples, and future generations. Justice also calls for accountability from those historically responsible for the majority of emissions, primarily developed countries and large corporations.
Who Bears Responsibility? Countries, Corporations, Rich vs. Poor
Countries: Responsibility for climate change is often divided between developed and developing nations. Developed countries, having contributed the most to historical emissions, are seen as having greater responsibility for leading mitigation efforts and providing financial and technological support to developing nations.
Corporations: Large corporations, particularly those in fossil fuel, energy-intensive industries, and major consumer brands, bear significant responsibility for emissions. There is increasing pressure on these corporations to reduce their carbon footprints, disclose climate risks, and invest in sustainable practices.
Rich vs. Poor: Wealthy individuals and countries have higher per capita emissions and more resources to invest in mitigation and adaptation. In contrast, poorer communities and nations are often the most vulnerable to climate impacts but have contributed the least to the problem. This disparity raises questions of equity and fairness in climate action.
The Emissions Gap
The emissions gap refers to the difference between the emissions reductions pledged by countries (NDCs) and the reductions needed to keep global warming below the 2ยฐC or 1.5ยฐC targets. Current pledges are insufficient, leading to a gap that needs to be addressed through enhanced ambition and accelerated action.
Implications: Closing the emissions gap requires urgent and significant increases in mitigation efforts globally. This includes transitioning to renewable energy, improving energy efficiency, protecting forests, and adopting sustainable agricultural practices. Failure to close the gap will result in more severe climate impacts and higher adaptation costs.
Grassroots Climate Justice Movements
Grassroots movements play a crucial role in advocating for climate justice and pushing for stronger climate action. These movements often emerge from communities directly affected by climate change and environmental degradation, emphasizing local solutions and inclusive decision-making.
Examples: Notable grassroots movements include Fridays for Future, led by young climate activists like Greta Thunberg; the Indigenous Environmental Network, advocating for the rights of indigenous peoples; and Extinction Rebellion, which uses nonviolent civil disobedience to demand urgent action.
Lawsuits
Climate-related lawsuits have been filed against governments and corporations for failing to adequately address climate change. These cases often seek to enforce existing environmental laws, challenge inadequate climate policies, or hold polluters accountable for damages.
Notable Examples: Landmark cases include Massachusetts v. EPA (2007), where the U.S. Supreme Court ruled that the EPA must regulate greenhouse gases; Urgenda Foundation v. State of the Netherlands (2019), where the Dutch Supreme Court ordered the government to reduce emissions by at least 25% by 2020; and Juliana v. United States, a youth-led lawsuit asserting a constitutional right to a stable climate.
Individual Action
Impact: While systemic change is essential, individual actions can collectively make a significant impact on emissions. This includes reducing energy consumption, adopting sustainable diets, using public transportation, and supporting green products and companies.
Advocacy: Individuals can also influence broader change by advocating for stronger climate policies, participating in climate movements, and holding leaders and corporations accountable. Education and raising awareness are critical components of driving societal shifts towards sustainability.
Cleaning Up Electricity
Energy Transitions in Different Contexts
Energy transitions refer to the shift from fossil fuel-based energy systems to cleaner, renewable energy sources. These transitions occur differently across various regions and contexts, influenced by factors such as economic development, resource availability, and political landscape.
Developed Countries: In many developed nations, the focus is on upgrading aging infrastructure, increasing the share of renewables, and enhancing energy efficiency. Countries like Germany and Denmark have made significant strides in integrating wind and solar power, often supported by strong policy frameworks and public investment.
Developing Countries: Developing nations face the dual challenge of meeting growing energy demand while transitioning to cleaner sources. Many of these countries have abundant renewable resources and can leapfrog directly to cleaner technologies. For example, India has ambitious targets for solar energy, and several African countries are exploring geothermal and hydroelectric power.
Resource-Rich Countries: Countries with abundant fossil fuel resources, such as Saudi Arabia or Russia, face different dynamics. While there is potential for economic diversification through renewables, there are also significant economic and political barriers due to the existing reliance on fossil fuel revenues.
Isolated and Rural Areas: In isolated or rural areas, decentralized energy solutions like solar home systems, microgrids, and small-scale wind or hydro installations can be more viable than extending central grid infrastructure. These solutions provide reliable electricity access and can be pivotal for economic development.
Clean Energy Technology Solutions
Transitioning to clean electricity involves deploying a range of technologies to replace or supplement fossil fuels. Key clean energy technologies include:
Solar Power: Photovoltaic (PV) systems and concentrated solar power (CSP) are the primary technologies. Solar power is scalable, from rooftop panels to large solar farms. Advances in efficiency and storage are making solar increasingly competitive.
Wind Power: Both onshore and offshore wind turbines are vital components of clean energy strategies. Offshore wind, in particular, has vast potential due to stronger and more consistent wind resources.
Hydropower: Large-scale hydropower has long been a major renewable source, but small-scale and run-of-the-river projects are gaining attention for their lower environmental impact.
Geothermal Energy: Exploiting the Earth's heat for electricity and heating. Geothermal plants provide a stable and reliable power source but are geographically limited to regions with suitable geological conditions.
Biomass and Bioenergy: Using organic materials for energy can be sustainable if managed properly. Technologies include biofuels for transportation and biogas for electricity and heating.
Energy Storage: Batteries, pumped hydro storage, and other storage technologies are crucial for balancing intermittent renewable sources like solar and wind. Advances in lithium-ion and emerging technologies like solid-state batteries are critical for grid stability and renewable integration.
Smart Grids: Modernizing electricity grids with digital technology to enhance reliability, efficiency, and integration of distributed renewable energy sources. Smart grids enable better demand response and energy management.
Energy Markets, Policy, and Regulations
Effective energy transitions require supportive markets, policies, and regulatory frameworks:
Energy Markets: Competitive energy markets can drive innovation and cost reduction in clean energy technologies. Policies that facilitate renewable energy integration and incentivize investment are crucial. Examples include feed-in tariffs, renewable energy certificates, and auctions for renewable projects.
Policy Instruments: Governments use various policy tools to promote clean energy, including subsidies, tax incentives, and grants for research and development. Regulatory measures such as renewable portfolio standards (RPS) mandate a specific share of renewables in the energy mix.
Carbon Pricing: Implementing carbon taxes or cap-and-trade systems to internalize the environmental costs of carbon emissions, thereby making renewable energy more competitive relative to fossil fuels.
Grid Regulations: Modernizing grid codes to support renewable integration, ensuring reliable grid operations, and facilitating cross-border electricity trade where feasible.
Political and Justice Considerations
Political and justice considerations are central to ensuring that the transition to clean energy is equitable and inclusive:
Political Will and Leadership: Strong political commitment and leadership are essential for driving energy transitions. This includes setting ambitious targets, securing public and private investment, and fostering international cooperation.
Economic Justice: Ensuring that the benefits of clean energy transitions are widely shared and do not disproportionately burden disadvantaged communities. This involves creating green jobs, supporting workers transitioning from fossil fuel industries, and providing affordable clean energy access.
Environmental Justice: Addressing the disproportionate environmental impacts of energy projects on marginalized communities. This includes ensuring that renewable energy projects do not replicate the harms of fossil fuel extraction, such as land displacement and environmental degradation.
Energy Access: Prioritizing energy access for all, particularly in underserved and remote areas. Clean energy can provide a reliable and sustainable solution to energy poverty, improving health, education, and economic opportunities.
Community Involvement: Engaging local communities in decision-making processes for energy projects to ensure that their needs and perspectives are considered. This can enhance project acceptance and success.
In summary, cleaning up electricity involves a complex interplay of technology, policy, market dynamics, and socio-political factors. A successful transition requires coordinated efforts across multiple sectors, with a strong emphasis on equity and justice to ensure that the benefits of clean energy are accessible to all.
Emission Reduction
The Electrification Imperative
The world is facing a critical juncture. Climate change demands a significant reduction in greenhouse gas emissions, and electrification presents a powerful solution. This approach involves replacing fossil fuel-powered technologies with electric alternatives across various sectors. While challenges exist, the potential benefits โ reduced emissions, improved air quality, and increased efficiency โ make electrification a compelling strategy for a sustainable future.
Decarbonizing Transportation: A Multifaceted Approach
Transportation is a major culprit behind greenhouse gas emissions. Effectively decarbonizing this sector requires a comprehensive strategy that considers different aspects:
Identifying Emission Sources: Understanding the primary contributors is crucial. Passenger cars, especially gasoline-powered ones, are a major source of urban emissions. Diesel trucks and airplanes also contribute significantly.
Promoting Sustainable Urban Mobility: Encouraging public transportation, cycling, and walkable cities can significantly reduce reliance on private vehicles. This not only cuts emissions but also fosters healthier lifestyles.
Electrifying Vehicles: Shifting towards electric cars, buses, two-wheelers, and even short-haul trucks is essential. Investment in charging infrastructure is crucial to enable a smooth transition.
Electrification for Long-Haul Transport: While electrifying long-haul trucking and airplanes might require technological advancements, solutions exist for the interim. Biofuels can be a temporary option for long-distance trucking, and electrification of short-haul ferries is a promising approach. For large cargo ships, exploring alternative fuels like ammonia or hydrogen fuel cells holds promise.
Transforming Buildings: Efficiency and Clean Energy
Buildings are responsible for a substantial portion of global energy consumption and emissions. Decarbonizing this sector requires a multi-pronged approach:
Understanding Building Emissions: Identifying the main culprits within buildings is the first step. Heating and cooling systems, appliances, and air conditioning are major contributors.
Economical Solutions: Focusing on cost-effective measures like energy-efficient windows, insulation, and LED lighting can significantly reduce energy use without breaking the bank. Governments and institutions can incentivize these upgrades through tax breaks or rebates.
Showcasing Success: High-profile examples of energy-efficient buildings can serve as powerful motivators. By demonstrating the economic and environmental benefits of sustainable building practices, a wave of change can be encouraged.
Heat Pumps and Cool Roofs: Promoting electric heat pumps for heating and cooling offers a clean alternative to fossil fuel-based systems. Additionally, encouraging reflective roofs that absorb less heat can significantly reduce energy demand for cooling, particularly in warmer climates.
Addressing Global Household Cooking: Millions of people worldwide rely on traditional biomass cooking methods, often involving wood-burning stoves. These contribute significantly to air pollution and health problems. Providing access to clean and efficient electric cooking solutions is essential for improving public health and reducing emissions.
A systems approach is necessary for holistic building decarbonization. Building design, energy-efficient technologies, and the integration of renewable energy sources for powering these buildings are all crucial aspects that need to work together seamlessly.
Industry Transformation: Electrification and Beyond
Industrial processes are another major source of greenhouse gas emissions. While electrification offers opportunities for some sectors, a multifaceted approach is needed:
Identifying Industrial Emitters: Steel production, cement manufacturing, and chemical production are some of the biggest emitters. Tailoring solutions to these specific industries is crucial.
Electrification Potential: Focusing on electrifying processes wherever possible is a significant step. For instance, high-temperature heat pumps show promise for industrial applications.
Transforming Steel Production: Transitioning to electric arc furnaces powered by renewable energy for steel production can significantly reduce emissions compared to traditional methods.
Rethinking Nitrogen Fertilizer Production: The Haber-Bosch process, the traditional method for nitrogen fixation, is energy-intensive. Exploring alternative methods for creating fertilizers is essential.
Sustainable Plastics: Research on bioplastics and closed-loop recycling processes can dramatically reduce reliance on fossil fuel-derived plastics, a significant source of emissions.
Exploring Alternative Fuels: For certain industries where electrification might not be feasible in the near future, exploring alternative fuels like hydrogen can be a viable option.
Regenerative Agriculture and Climate Change
Regenerative agriculture impacts climate change through several key mechanisms: carbon sequestration, reduction of greenhouse gas emissions, and increased resilience to climate impacts. Hereโs a detailed look at each of these mechanisms:
1. Carbon Sequestration
Soil Carbon Storage:
Soil Organic Matter: Regenerative practices enhance soil organic matter, which is a significant carbon sink. Techniques such as cover cropping, crop rotation, and reduced tillage increase the amount of organic material in the soil, promoting carbon storage.
Root Systems: Deep-rooted plants and perennials used in regenerative farming improve soil structure and carbon sequestration. The roots of these plants deposit carbon deeper into the soil, where it can remain stored for longer periods.
Agroforestry:
Trees and Shrubs: Integrating trees and shrubs into agricultural landscapes increases biomass above and below ground, enhancing carbon storage. Trees capture CO2 from the atmosphere and store it in their biomass and the soil.
Perennial Systems: Using perennial crops and polycultures in agroforestry systems ensures continuous carbon capture and reduces soil disturbance.
2. Reduction of Greenhouse Gas Emissions
Reduced Reliance on Chemical Inputs:
Fertilizers: Synthetic nitrogen fertilizers are a major source of nitrous oxide (N2O), a potent greenhouse gas. Regenerative practices that use organic fertilizers, compost, and nitrogen-fixing cover crops reduce the need for synthetic fertilizers, thereby lowering N2O emissions.
Pesticides and Herbicides: Reduced use of chemical pesticides and herbicides in regenerative systems decreases emissions associated with their production and application.
Improved Livestock Management:
Managed Grazing: Practices such as rotational grazing improve pasture health, enhance soil carbon sequestration, and reduce methane (CH4) emissions from livestock. Healthy pastures lead to better digestion in ruminants, potentially lowering methane emissions.
Manure Management: Using manure as a fertilizer in a controlled manner reduces methane emissions from manure decomposition and enhances soil health.
Enhanced Energy Efficiency:
Reduced Tillage: Minimizing soil disturbance reduces fuel use for machinery and lowers CO2 emissions from agricultural operations.
Local Food Systems: Regenerative agriculture often emphasizes local food production, reducing the carbon footprint associated with transportation and distribution of food.
3. Increased Resilience to Climate Impacts
Soil Health and Water Retention:
Water Infiltration: Healthy soils with high organic matter content have better water infiltration and retention, making crops more resilient to droughts and reducing the need for irrigation.
Erosion Control: Practices like cover cropping and maintaining permanent soil cover reduce soil erosion, preserving topsoil and maintaining productivity during extreme weather events.
Biodiversity and Ecosystem Services:
Pest and Disease Control: Biodiverse farming systems are more resilient to pests and diseases, reducing the need for chemical interventions and enhancing ecosystem stability.
Pollination and Soil Health: Increased biodiversity supports pollinators and soil organisms, which are crucial for plant health and productivity, further enhancing resilience.
Quantitative Impact
Carbon Sequestration Potential:
Estimates suggest that regenerative practices can sequester between 0.5 to 2.5 metric tons of CO2 per hectare per year, depending on the specific practices and local conditions.
Scaling these practices globally could significantly contribute to climate mitigation efforts, potentially offsetting a substantial portion of global CO2 emissions.
Emission Reductions:
Transitioning to regenerative systems can reduce agricultural greenhouse gas emissions by improving efficiency and reducing the need for synthetic inputs. The magnitude of these reductions varies widely but can be significant at the farm level.
Food Supply Chains and Decarbonization
Decarbonizing food supply chains involves reducing greenhouse gas (GHG) emissions at every stage of the food production, processing, distribution, consumption, and waste management cycle.
1. Agricultural Production
Sustainable Farming Practices:
Regenerative Agriculture: Practices like cover cropping, reduced tillage, and agroforestry enhance soil health and carbon sequestration.
Organic Farming: Reduces reliance on synthetic fertilizers and pesticides, lowering emissions from their production and application.
Precision Agriculture: Utilizes technology to optimize inputs (water, fertilizers, pesticides) and improve efficiency, reducing emissions.
Alternative Protein Sources:
Plant-Based Proteins: Shifting from animal-based proteins to plant-based proteins (e.g., legumes, soy, and quinoa) can significantly reduce GHG emissions.
Lab-Grown Meat: Cultured meat production has the potential to produce fewer emissions compared to traditional livestock farming.
Livestock Management:
Improved Feed: Enhancing livestock diets to improve digestion and reduce methane emissions.
Manure Management: Efficient management and use of manure as fertilizer to reduce methane and nitrous oxide emissions.
2. Processing and Packaging
Energy Efficiency:
Renewable Energy: Utilizing solar, wind, or biogas energy in food processing facilities to reduce reliance on fossil fuels.
Energy-Efficient Technologies: Upgrading energy-efficient equipment and optimizing production processes.
Sustainable Packaging:
Biodegradable Materials: Using biodegradable and compostable packaging materials to reduce waste and emissions.
Recyclable Packaging: Designing packaging for easy recycling to reduce the carbon footprint of packaging materials.
Reducing Food Waste:
Efficient Processing: Improving processing techniques to minimize food waste.
Byproduct Utilization: Converting food byproducts into useful products (e.g., animal feed, bioenergy).
3. Distribution
Transportation:
Low-Emission Vehicles: Transitioning to electric or hydrogen-powered trucks and ships for food transportation.
Efficient Logistics: Optimizing supply chain logistics to reduce transportation distances and improve fuel efficiency.
Local Sourcing:
Shorter Supply Chains: Sourcing food locally to reduce transportation emissions and support regional economies.
Urban Farming: Promoting urban agriculture to reduce the distance food travels from farm to table.
4. Retail and Consumption
Energy Management:
Efficient Refrigeration: Using energy-efficient refrigeration and HVAC systems in retail stores to reduce energy consumption.
Renewable Energy: Installing solar panels and other renewable energy sources in retail facilities.
Consumer Behavior:
Awareness Campaigns: Educating consumers about the carbon footprint of their food choices and encouraging sustainable consumption.
Sustainable Diets: Promoting diets with lower environmental impacts, such as plant-based and locally sourced foods.
Food Waste Reduction:
Waste Minimization: Implementing strategies to reduce food waste at the retail level, such as better inventory management and donation of unsold food.
Consumer Practices: Encouraging consumers to plan meals, store food properly, and use leftovers to reduce household food waste.
5. Waste Management
Organic Waste Recycling:
Composting: Diverting food waste from landfills to composting facilities to produce organic fertilizer and reduce methane emissions.
Anaerobic Digestion: Using anaerobic digestion to convert organic waste into biogas and digestate, which can be used as renewable energy and fertilizer.
Circular Economy:
Resource Recovery: Implementing systems to recover and reuse nutrients and energy from food waste.
Zero-Waste Initiatives: Developing policies and practices aimed at achieving zero waste in food supply chains.
Carbon Removal
Climate change is a complex challenge demanding a multifaceted response. While curbing greenhouse gas emissions remains paramount, carbon removal offers an additional strategy to combat climate change. These techniques actively capture and store carbon dioxide (CO2) already in the atmosphere, helping to mitigate the effects of greenhouse gas emissions and buy us valuable time as we transition towards a low-carbon economy.
The Science of Carbon Sequestration:
Carbon removal relies on the concept of carbon sequestration, the long-term storage of CO2 in various reservoirs. There are two main approaches, each with its advantages and considerations:
1. Natural Carbon Removal: This leverages the inherent ability of natural ecosystems to absorb and store CO2. Forests, wetlands, agricultural lands, and even oceans all play a vital role in the global carbon cycle. By enhancing these natural processes, we can significantly increase the amount of CO2 removed from the atmosphere.
2. Technology-Based Carbon Removal: This utilizes engineered solutions to capture CO2 directly from the atmosphere. These technologies are still under development, but they offer the potential for large-scale CO2 removal and can be particularly useful for addressing emissions that are difficult to mitigate at the source, such as those from industrial processes.
Natural Carbon Removal: Working with Nature's Infrastructure
Forests as Carbon Powerhouses: Forests are Earth's champions for carbon capture. Trees absorb CO2 through photosynthesis and store it in their biomass (trunks, branches, and leaves). Protecting existing forests from deforestation and degradation is vital. Planting new trees (afforestation) and promoting sustainable forestry practices that avoid clearcutting and prioritize native species are all essential for maximizing natural CO2 capture.
Wetlands: Nature's Sponges for Carbon Storage: Wetlands, like bogs and marshes, are surprisingly effective at carbon storage. They contain saturated soils rich in organic matter that can store vast amounts of CO2. Protecting and restoring wetlands can significantly enhance their role in mitigating climate change.
Agricultural Practices that Enhance Soil Carbon: Certain agricultural practices can promote carbon sequestration in soil, improving soil health and fertility in the process. These practices include reducing tillage (disturbing the soil), using cover crops (plants grown between cash crops to suppress weeds and improve soil health), and applying biochar (a charcoal-like substance produced by heating organic matter). These methods help trap carbon dioxide in the soil, reducing reliance on chemical fertilizers and creating a more sustainable agricultural system.
Oceans: The Vast Blue Carbon Reservoir: Oceans play an irreplaceable role in the global carbon cycle, absorbing a significant portion of atmospheric CO2. Phytoplankton (microscopic marine plants) capture CO2 during photosynthesis. When they die and sink to the ocean floor, some of the carbon gets buried in sediments, storing it away for long periods. Protecting and restoring coastal ecosystems like mangroves and seagrasses can further enhance the ocean's capacity for carbon storage.
Technology-Based Carbon Removal:
Direct Air Capture (DAC): DAC technology uses machines equipped with special filters or chemical reactions to capture CO2 directly from the air. These machines operate like giant air filters, selectively removing CO2 molecules from the ambient air. The captured CO2 can then be stored underground in geological formations like saline aquifers (deep underground rock formations containing salty water) or utilized for various purposes like producing synthetic fuels. While promising, DAC is still under development and requires significant energy input for operation. Research is ongoing to improve efficiency and reduce the costs of DAC technologies.
Carbon Capture and Storage (CCS): This approach focuses on capturing CO2 emissions from industrial sources like power plants or factories before they are released into the atmosphere. The captured CO2 is then transported, often via pipelines, and injected into suitable geological formations for permanent storage. CCS can be a valuable tool for reducing emissions from industries that are difficult to decarbonize completely, but it raises concerns about potential leakage of stored CO2 and the long-term safety of storage sites.
Bioenergy with Carbon Capture and Storage (BECCS): This combines biomass production with CCS technology. BECCS involves growing biomass (plants), converting it into biofuels, and capturing the CO2 emissions generated during the conversion process. The captured CO2 is then stored underground, creating a negative emissions technology โ effectively removing CO2 from the atmosphere. However, BECCS also raises concerns about land-use change and the sustainability of large-scale biomass production.
Carbon Offsets
In the fight against climate change, carbon offsets have emerged as a tool for individuals and organizations to compensate for their carbon footprint. Let's delve deeper into the concept, explore the complexities involved, and consider the future of offsets within the broader climate action landscape.
Understanding Carbon Offsets: Balancing Your Carbon Footprint
Imagine you take a flight, generating greenhouse gas emissions. A carbon offset allows you to invest in projects that reduce, avoid, or remove an equivalent amount of CO2 from the atmosphere elsewhere. This could involve planting trees in a degraded forest, supporting the development of a solar power plant in a developing country, or capturing emissions from an industrial facility.
By purchasing offsets, you essentially contribute to projects that counteract the emissions you can't readily avoid in your daily life. This creates a balance sheet for your carbon footprint, aiming for net-zero emissions. Offsets can be particularly relevant for activities that are difficult to decarbonize entirely, like air travel or certain industrial processes.
Challenges and Complexities: Ensuring Credibility in the Offset Market
While offsets offer a seemingly straightforward solution, several challenges need to be addressed to ensure their effectiveness and credibility:
Additionality: A critical question is whether the offset project would have happened anyway, even without the offset funding. Ideally, offsets should support projects that wouldn't exist otherwise, creating additional emission reductions. Without additionality, offsets wouldn't be truly mitigating new emissions, but simply paying for existing reductions.
Permanence: Certain offset projects, like tree planting, carry the risk of reversal. Fires, natural disasters, or even changes in land use can release stored carbon back into the atmosphere. Ensuring the permanence of carbon sequestration is crucial. Reforestation projects, for instance, need to be carefully planned and managed to ensure the long-term survival of the trees.
Measurement and Verification (MRV): Accurately measuring the amount of CO2 removed or avoided by offset projects is complex. Robust verification processes are essential to ensure the integrity of the offset market. Independent third-party verification bodies play a vital role in ensuring that offset projects deliver the promised emission reductions.
Transparency and Quality: The current offset market can lack complete transparency. Buyers need clear information about the project type, location, the methodologies used to calculate emission reductions, and the potential risks associated with the project. Reputable offset providers should be able to furnish buyers with detailed information about the projects they support.
The Future of Carbon Offsets: Building Trust and Addressing Challenges
Despite these challenges, the future of carbon offsets isn't without hope. Here's a glimpse into what's on the horizon for the carbon offset market:
Improved Standards: Standardization bodies are developing stricter criteria to ensure the quality and credibility of offset projects. These standards will help ensure that offsets represent real, measurable, and additional emission reductions.
Technological Advancements: New technologies, like satellite monitoring for forestry projects, are improving the measurement, reporting, and verification (MRV) of offsets. These advancements will enable more accurate assessment of the carbon sequestration potential of various offset projects.
Focus on Permanence: Projects that prioritize permanent carbon storage, such as geologic storage of captured CO2, are gaining traction. These projects offer a more permanent solution to mitigating climate change compared to offsets relying on biological processes like tree planting.
Evolving Market Mechanisms: Offset markets are evolving to include innovative project types and explore new technologies for carbon removal. This will broaden the range of options available to offset buyers and potentially lower the costs associated with high-quality offsets.
Beyond Offsets: A Hierarchy of Climate Action
It's important to remember that carbon offsets should be considered a last resort in a climate action hierarchy. Here's the ideal order of action:
1. Reduction: The primary focus should be on reducing your own carbon footprint through energy efficiency, sustainable transportation choices, and conscious consumption. This involves making changes in your daily life to minimize the amount of CO2 emissions you generate in the first place.
2. Removal: Supporting technologies like direct air capture or BECCS (Bioenergy with Carbon Capture and Storage) can directly remove existing CO2 from the atmosphere. These technologies offer a more proactive approach to combating climate change by actively removing greenhouse gases instead of simply offsetting them.
3. Offsets: If emissions are unavoidable, then consider high-quality, verified carbon offsets as a way to compensate for your remaining footprint. Look for offsets that meet stringent standards, prioritize permanence, and offer clear information about the project and its impact.
Public Green Finance
Public green finance stands as a crucial pillar in the fight against climate change. It encompasses the various financial instruments and strategies employed by governments and multilateral institutions to promote climate-friendly activities. Let's delve deeper into the specific approaches adopted by the US, India, multilateral organizations, and the innovative concept of debt-for-climate swaps.
The US Green Finance Landscape:
The United States plays a significant role in global climate finance. While the US approach might differ from some other nations, here are key instruments used to spur clean investments:
Tax Incentives: The US government offers tax breaks for businesses and individuals who invest in renewable energy projects like solar and wind power installations. These tax breaks can significantly reduce the upfront cost of clean technologies, making them more attractive investments.
Loan Guarantees: Government-backed loan guarantees provide a safety net for lenders financing clean technology projects. This reduces the perceived risk for lenders, encouraging them to provide loans for these projects at more favorable rates.
Research and Development (R&D) Funding: The US government invests in research and development of clean technologies, fostering innovation in areas like energy storage, carbon capture, and advanced biofuels. This helps accelerate the development and deployment of these technologies.
Federal Grant Programs: Several federal grant programs exist to support climate action initiatives. These grants can be used for various purposes, such as developing climate resilience plans for communities, supporting energy efficiency upgrades in buildings, or promoting sustainable transportation solutions.
India's Green Ambition:
India has emerged as a leader in the global green finance movement. Here's a closer look at its strategies:
Renewable Energy Targets: India has set ambitious renewable energy targets, aiming for a significant portion of its power generation to come from clean sources like solar and wind. This creates a strong market for renewable energy technologies, attracting investments in solar panel manufacturing, wind turbine installations, and grid modernization projects.
Subsidies for Clean Technologies: The Indian government offers subsidies for electric vehicles and green infrastructure development. This helps reduce the upfront costs for consumers and businesses, making clean technologies more accessible and fostering their adoption.
Green Bonds: India has actively issued green bonds to raise funds specifically for climate-friendly projects. These bonds appeal to investors seeking a combination of financial returns and positive environmental impact.
National Green Hydrogen Mission: India has launched a national mission to develop green hydrogen, a clean fuel produced by splitting water using renewable energy. This mission aims to create a domestic green hydrogen industry and reduce reliance on fossil fuels.
Multilateral Institutions: A Global Response
International organizations play a vital role in mobilizing climate finance for developing countries. Here are two key players:
The World Bank: The World Bank provides loans, grants, and technical assistance to developing countries for climate action projects. These projects can encompass renewable energy development, climate-smart agriculture practices, and building climate resilience in vulnerable communities.
The Green Climate Fund (GCF): The GCF is a dedicated multilateral fund that supports developing countries in their efforts to respond to climate change. It provides financing for adaptation and mitigation projects, with a focus on the needs of the most vulnerable countries.
Debt-for-Climate Swaps: An Innovative Approach
Debt-for-climate swaps offer a creative solution to address both climate change and developing country debt. Here's how it works:
Debt Relief for Sustainability: A creditor nation (developed country) agrees to forgive a portion of a developing country's debt.
Commitment to Green Initiatives: In exchange for debt relief, the developing country agrees to allocate the freed-up resources towards environmental projects. These projects could involve forest conservation, renewable energy development, or climate-resilient infrastructure development.
This approach provides developing countries with much-needed financial resources for environmental initiatives while simultaneously reducing their debt burden. However, careful design and implementation are crucial to ensure the effectiveness of debt-for-climate swaps.
Private Green Finance
Public green finance plays a vital role, but the fight against climate change also requires the muscle of the private sector. Private green finance encompasses various financial instruments and strategies employed by private investors to support climate-friendly activities and generate positive environmental impact alongside financial returns.
ESG Investing: A Values-Driven Approach
Environmental, Social, and Governance (ESG) factors: This approach integrates the consideration of environmental, social, and governance factors alongside traditional financial metrics when making investment decisions. Investors consider a company's environmental footprint, its social responsibility practices, and its corporate governance structure to assess its long-term sustainability and potential risks.
Benefits of ESG Investing: By investing in companies with strong ESG practices, investors can contribute to a more sustainable future while potentially mitigating risks associated with environmental regulations, social unrest, or poor corporate governance. Studies also suggest that companies with strong ESG performance might outperform in the long run.
Green Bonds: Financing the Green Transition
Green Bonds Explained: Green bonds are fixed-income securities issued by corporations, governments, or international organizations to raise capital specifically for climate-friendly projects. These projects can encompass renewable energy infrastructure, energy efficiency improvements, sustainable transportation, or green buildings.
The Greenium Effect: Green bonds often attract investors seeking both financial returns and positive environmental impact. This can sometimes lead to a "greenium effect," where green bonds offer slightly lower interest rates than traditional bonds due to higher investor demand.
Transparency and Standards: The green bond market relies on clear definitions and reporting standards to ensure the legitimacy and impact of labeled projects. Several organizations issue green bond principles and certifications to provide transparency and investor confidence.
Climate Tech VC: Fueling Innovation
Climate Tech Defined: Climate tech refers to innovative technologies designed to address climate change. This encompasses a wide range of solutions, from renewable energy generation and energy storage to carbon capture and sustainable materials.
Venture Capital (VC) Firms: VC firms invest in early-stage, high-growth companies. Climate tech VC firms focus on supporting promising startups developing solutions for climate change. This provides crucial seed funding for these emerging technologies to mature and potentially reach commercial scale.
The Risk-Reward Equation: Investing in early-stage companies inherently carries a high degree of risk. However, climate tech VC firms see the potential for significant long-term returns alongside the environmental benefits of the technologies they support.
Beyond the Basics: Other Avenues in Private Green Finance
The private green finance landscape extends beyond these core areas. Here are some additional noteworthy trends:
Green Insurance: Insurance companies are increasingly offering products that incentivize sustainable practices or provide coverage for climate-related risks.
Impact Investing: This growing investment approach focuses on generating social and environmental impact alongside financial returns. Climate change mitigation and adaptation are key themes within impact investing.
Green Real Estate: Investments in energy-efficient buildings, sustainable construction practices, and green infrastructure development are gaining traction.
Challenges and Opportunities: The Future of Private Green Finance
While private green finance offers immense potential, there are challenges to address:
Standardization and Transparency: Ensuring clear definitions and consistent reporting methods for green investments remains crucial.
Risk Management: Developing robust frameworks to assess and manage environmental risks associated with investments is essential.
Long-Term Investor Mindset: Shifting investor focus towards long-term sustainability goals alongside immediate returns is crucial for sustained growth of private green finance.
Blended Green Finance
Climate action demands significant investments, but traditional financial resources often fall short, particularly for high-impact projects in developing countries. Blended green finance emerges as a strategic solution, bringing together public and private resources to make climate-friendly projects more attractive and financially viable. Here's a deeper dive into this innovative approach:
Bridging the Gap Between Public and Private Finance
Public Funds as a Catalyst: Public resources from governments, multilateral institutions, or philanthropic organizations play a crucial role in blended green finance. These funds can be used in various ways, such as:
Providing concessional loans: Loans with lower interest rates or longer repayment periods can make projects more affordable for private investors.
Offering grants or equity investments: Public funds can directly invest in projects, reducing the upfront risk for private investors.
Providing guarantees against risks: Public guarantees can mitigate perceived risks associated with climate projects, encouraging private sector participation.
Private Capital for Scale: Blended finance aims to leverage public funds to attract significant private investments. By making projects more attractive and reducing risk, public resources can unlock much larger pools of private capital. This allows for scaling up climate action initiatives and achieving greater impact.
Examples of Blended Green Finance in Action
Blended finance can be applied across various climate-related sectors. Here are a few illustrations:
Renewable Energy in Developing Countries: Public funds can be used to de-risk renewable energy projects in developing countries, making them more attractive for private investors. This can lead to increased investment in solar, wind, or geothermal energy projects, accelerating the transition away from fossil fuels.
Climate-Smart Agriculture: Public grants or guarantees can support projects promoting sustainable agricultural practices that reduce greenhouse gas emissions and improve soil health. This can incentivize private sector involvement in developing and deploying these approaches.
Building Climate Resilience in Vulnerable Communities: Public funds can be combined with private investments to finance disaster risk reduction infrastructure or climate-resilient housing projects in areas vulnerable to climate change impacts.
Benefits of Blended Green Finance:
Mobilizing Large-Scale Investments: Blended finance allows for mobilizing significantly more capital for climate action than either public or private resources could achieve alone.
Reduced Risk for Private Investors: Public involvement helps mitigate risks associated with climate projects, making them more attractive for private investment.
Improved Project Design and Implementation: Collaboration between public and private stakeholders can lead to more robust project development, implementation, and monitoring.
Challenges and Considerations
While blended finance offers immense promise, some challenges need to be addressed:
Complexity and Transaction Costs: Structuring blended finance deals can be complex and time-consuming, requiring expertise to navigate different funding streams and regulations.
Project Selection and Impact Measurement: Ensuring project selection prioritizes genuine climate impact and aligning the interests of public and private stakeholders requires careful consideration.
Long-Term Sustainability: The long-term financial sustainability and impact of blended finance projects need to be carefully evaluated.
Climate Risks
Climate change introduces significant risks to the financial sector, impacting asset values, market stability, and economic performance. These risks are categorized into physical risks, transition risks, and stranded assets, each affecting the financial sector in unique ways. Financial institutions, including banks, insurers, and asset managers, must identify, assess, and manage these risks to safeguard their portfolios and ensure sustainable financial practices.
Physical Climate Risks
Physical climate risks are the direct impacts of climate change on physical assets, categorized into acute and chronic risks.
1. Acute Risks:
Extreme Weather Events: Hurricanes, floods, wildfires, and heatwaves can cause immediate and catastrophic damage to infrastructure, real estate, agricultural outputs, and supply chains. For example, Hurricane Katrina in 2005 caused over $125 billion in damages, highlighting the significant financial implications of such events.
Insurance Claims: These events result in increased insurance claims, affecting the profitability and solvency of insurance companies. For instance, the wildfire season in California has led to billions in insurance claims, challenging the sector's capacity to underwrite such risks.
2. Chronic Risks:
Long-term Environmental Changes: Gradual changes such as rising sea levels, increasing temperatures, and changing precipitation patterns can degrade asset values over time. Coastal properties may face devaluation due to increased flood risks, and agricultural yields may decline due to altered growing conditions.
Operational Costs: Businesses may incur higher operational costs due to the need for climate adaptation measures, such as investing in flood defenses or cooling systems for heat-sensitive operations.
Transition Risks
Transition risks emerge from the global shift towards a low-carbon economy, driven by policy changes, technological advancements, and evolving market preferences.
1. Policy and Regulatory Changes:
Carbon Pricing: Implementation of carbon taxes or cap-and-trade systems increases operational costs for carbon-intensive industries. The EU Emissions Trading System (ETS), for example, has led to significant financial impacts on heavy industries.
Regulations and Standards: Stricter environmental regulations can mandate emissions reductions, energy efficiency standards, and renewable energy adoption, impacting the profitability of companies unable to adapt swiftly.
2. Technological Advancements:
Renewable Energy Technologies: Innovations in solar, wind, and battery storage technologies are disrupting traditional energy markets. Companies investing in outdated technologies may face obsolescence and financial losses.
Energy Efficiency: Advances in energy-efficient technologies reduce demand for fossil fuels, affecting sectors like coal and oil.
3. Market and Consumer Preferences:
Sustainable Products: Increasing consumer demand for sustainable products pressures companies to adopt greener practices. Firms that fail to meet these expectations may suffer reputational damage and loss of market share.
Investor Preferences: Investors are increasingly incorporating ESG (Environmental, Social, and Governance) criteria into their investment decisions, favoring companies with strong sustainability profiles.
Stranded Assets
Stranded assets refer to investments that have lost their value due to the transition to a low-carbon economy. For example, fossil fuel reserves may become non-viable due to regulatory restrictions on carbon emissions, rendering these assets "stranded" and financially worthless.
Who Really Pays?
The financial burden of climate risks is distributed across various stakeholders:
1. Companies:
Direct Costs: Companies bear the immediate costs of physical damage from climate events, regulatory compliance costs, and expenses associated with transitioning to sustainable practices. For instance, energy companies may face significant costs to shift from fossil fuels to renewable energy sources.
Business Interruption: Disruptions in operations due to extreme weather events or supply chain interruptions can result in substantial financial losses.
2. Investors:
Portfolio Risk: Investors may experience reduced returns on investments in sectors or regions vulnerable to climate risks. For instance, real estate investments in coastal areas may depreciate due to rising sea levels.
Divestment: Investors might divest from high-risk industries, impacting stock prices and the ability of these companies to raise capital.
3. Consumers:
Price Increases: Companies often pass on the costs of climate adaptation and regulatory compliance to consumers, leading to higher prices for goods and services.
Insurance Premiums: Rising insurance premiums due to increased climate-related claims affect household budgets.
4. Governments and Taxpayers:
Disaster Relief and Infrastructure: Governments bear the costs of disaster relief, rebuilding infrastructure, and implementing climate adaptation measures. For example, the U.S. federal government spends billions annually on disaster response and recovery.
Public Health Costs: Climate change can exacerbate public health issues, leading to higher healthcare costs funded by taxpayers.
Financial Actors in Climate Risk Management
Several financial actors are critical in managing climate risks:
1. Banks and Lenders:
Credit Risk Assessment: Banks incorporate climate risk assessments into their credit evaluation processes, considering the potential impacts on borrowers' ability to repay loans. This includes evaluating the exposure of collateral to physical climate risks.
Green Financing: Banks are increasingly offering green loans and financing for projects that mitigate climate risks, such as renewable energy and energy efficiency projects.
2. Insurers:
Risk-Based Pricing: Insurers adjust premiums based on the assessed climate risks of insured assets. For instance, properties in high-risk flood zones face higher premiums.
New Insurance Products: Development of innovative insurance products to cover emerging climate risks, such as parametric insurance that provides payouts based on predefined weather conditions.
3. Asset Managers and Institutional Investors:
ESG Integration: Asset managers integrate ESG factors into their investment strategies to manage climate risks and identify sustainable investment opportunities. This includes screening for companies with robust climate risk management practices.
Active Ownership: Investors engage with companies to improve their climate risk management and sustainability practices, often through shareholder resolutions and voting.
4. Regulators and Central Banks:
Regulatory Frameworks: Regulators develop frameworks and guidelines to ensure financial institutions incorporate climate risks into their decision-making processes. For example, the Bank of England has introduced climate stress testing for banks.
Disclosure Requirements: Regulators may mandate disclosure of climate-related financial risks, enhancing transparency and informing investor decisions.
Evolving Regulatory Landscape
The regulatory landscape for climate risks in finance is evolving, with several key developments:
1. Disclosure Requirements:
TCFD: The Task Force on Climate-related Financial Disclosures (TCFD) provides recommendations for companies to disclose climate-related risks and opportunities. These include governance, strategy, risk management, and metrics and targets.
EU Taxonomy: The European Union has developed a taxonomy for sustainable activities, providing a classification system to help investors identify environmentally sustainable economic activities.
2. Stress Testing:
Scenario Analysis: Financial institutions are required to conduct scenario analysis to assess the resilience of their portfolios under different climate scenarios. This helps identify potential vulnerabilities and inform risk management strategies.
Regulatory Mandates: Central banks and financial regulators, such as the European Central Bank (ECB), are implementing climate stress tests to evaluate the impact of climate risks on financial stability.
3. Green Taxonomies:
Classification Systems: Green taxonomies define what constitutes environmentally sustainable activities, helping investors allocate capital to sustainable projects. The EU taxonomy is a prominent example, covering areas like climate change mitigation and adaptation.
4. Climate Risk Management Frameworks:
Guidelines and Standards: Regulatory bodies develop guidelines and standards for integrating climate risk assessments into financial decision-making. This includes risk management practices, governance structures, and reporting requirements.
Challenges
Managing climate risks in finance presents several challenges:
1. Data and Methodology:
Data Availability: Accurate climate risk assessment requires reliable data on physical and transition risks, which is often limited or inconsistent.
Methodological Challenges: Developing robust methodologies to quantify and integrate climate risks into financial models is complex and evolving.
2. Short-termism:
Focus on Short-term Returns: Financial markets often prioritize short-term returns, making it difficult to prioritize long-term climate risks. This can lead to underinvestment in climate resilience and sustainability.
3. Global Coordination:
Inconsistent Standards: Regulatory approaches to climate risks vary by country, leading to inconsistent standards and practices. This hampers global efforts to manage climate risks effectively.
Ways Forward
1. Enhanced Disclosure and Transparency:
Improving Quality and Consistency: Enhance the quality and consistency of climate-related disclosures to better inform investors and stakeholders. This includes standardizing reporting frameworks like TCFD and encouraging comprehensive risk assessments.
2. Collaborative Efforts:
Global Cooperation: Foster global cooperation among regulators, financial institutions, and governments to harmonize standards and share best practices. Initiatives like the Network for Greening the Financial System (NGFS) facilitate such collaboration.
3. Innovation in Financial Products:
Sustainable Investment Products: Develop and promote financial products that support sustainable investments, such as green bonds, climate-linked loans, and sustainability-linked bonds. These products incentivize companies to improve their environmental performance.
4. Capacity Building:
Training and Education: Equip financial institutions with the tools and expertise needed to assess and manage climate risks effectively. This includes training programs, research initiatives, and knowledge-sharing platforms.
Corporate Climate Action
Corporate Sustainability
Corporate sustainability involves incorporating environmental, social, and governance (ESG) considerations into business strategies to ensure long-term value creation. It is about balancing economic success with social equity and environmental protection.
1. Environmental Responsibility:
Resource Efficiency: Optimize the use of energy, water, and raw materials to reduce waste and lower costs. For example, manufacturing companies can implement energy-efficient technologies and processes to minimize energy consumption.
Waste Management: Implement waste reduction, recycling, and reuse programs. This includes managing hazardous waste safely and reducing landfill use.
Pollution Reduction: Adopt practices that reduce air, water, and soil pollution. This can include upgrading to cleaner production technologies and improving emission control systems.
2. Social Responsibility:
Labor Practices: Ensure fair wages, safe working conditions, and respect for workers' rights. Companies can adopt international labor standards such as those outlined by the International Labour Organization (ILO).
Community Engagement: Invest in local communities through education, health programs, and infrastructure development. This can enhance a companyโs reputation and build strong local relationships.
3. Economic Viability:
Long-term Planning: Focus on sustainable growth rather than short-term profits. This can involve investing in sustainable technologies and practices that offer long-term benefits.
Risk Management: Identify and mitigate risks related to environmental, social, and governance factors. This helps ensure the resilience and continuity of business operations.
ESG (Environmental, Social, and Governance)
ESG criteria are standards used by investors to evaluate companies' sustainability and ethical impact.
1. Environmental:
Climate Change Mitigation: Implement strategies to reduce greenhouse gas (GHG) emissions, such as energy efficiency measures and transitioning to renewable energy sources.
Sustainable Resource Management: Use resources like water, minerals, and forests sustainably. This includes reducing water consumption, preventing deforestation, and promoting sustainable agriculture.
2. Social:
Employee Welfare: Provide benefits, career development opportunities, and a safe working environment. This includes initiatives like health and wellness programs and continuous learning and development.
Community Development: Support local economies and social initiatives. This might involve partnerships with local non-profits or investments in local infrastructure projects.
3. Governance:
Board Diversity and Inclusion: Ensure a diverse and inclusive board of directors. Diversity in leadership can enhance decision-making and reflect the company's commitment to equality.
Transparency and Accountability: Maintain high standards of transparency in financial reporting and corporate practices. This involves clear communication with stakeholders and rigorous internal controls to prevent fraud and corruption.
Net-Zero Planning
Net-zero planning involves creating a strategy to achieve net-zero emissions, where the amount of emitted greenhouse gases is balanced by the amount removed from the atmosphere.
1. Setting Targets:
Science-Based Targets: Set targets in line with climate science and the Paris Agreement, aiming for a net-zero timeline, often by 2050 or sooner. This involves assessing the companyโs current emissions and identifying reduction pathways.
2. Emission Reduction:
Operational Changes: Implement energy-saving measures, optimize production processes, and switch to renewable energy sources. For instance, companies can upgrade their facilities to improve energy efficiency and reduce emissions.
Supply Chain Optimization: Work with suppliers to reduce emissions throughout the supply chain. This includes encouraging suppliers to adopt sustainable practices and improve their energy efficiency.
3. Carbon Offsetting:
Invest in Offsetting Projects: Support projects that remove or reduce carbon from the atmosphere, such as reforestation, renewable energy projects, and carbon capture and storage technologies. These projects can help offset emissions that cannot be eliminated.
Verified Carbon Credits: Purchase verified carbon credits from reputable sources to offset residual emissions. Ensure these credits are from projects that meet high environmental and social standards.
4. Monitoring and Reporting:
Regular Tracking: Continuously monitor and report on progress towards net-zero goals. Use tools and frameworks like the Greenhouse Gas Protocol for consistent tracking.
Transparency: Provide stakeholders with regular, transparent updates on emissions and reduction efforts. This can build trust and demonstrate the companyโs commitment to sustainability.
Life-Cycle Analysis (LCA)
Life-cycle analysis assesses the environmental impacts associated with all stages of a product's life, from raw material extraction to disposal.
1. Goal and Scope Definition:
Purpose: Define the objective of the LCA, such as reducing the environmental footprint of a product or improving resource efficiency.
Scope: Determine the boundaries of the study, including which life-cycle stages to assess and which environmental impacts to consider (e.g., carbon footprint, water use).
2. Inventory Analysis:
Data Collection: Gather data on all inputs (energy, materials) and outputs (emissions, waste) for each stage of the productโs life cycle.
Quantification: Quantify the inputs and outputs to create an inventory of the environmental flows.
3. Impact Assessment:
Impact Categories: Evaluate the potential environmental impacts in categories like global warming potential, acidification, and resource depletion.
Assessment Methods: Use established methods, such as TRACI (Tool for the Reduction and Assessment of Chemical and other environmental Impacts), to quantify impacts.
4. Interpretation:
Results Analysis: Analyze the results to identify key areas for improvement.
Recommendations: Provide actionable recommendations to reduce environmental impacts, such as material substitution or process improvements.
Managing Emissions
Managing emissions involves identifying and reducing greenhouse gas emissions across the organization.
1. Scope 1 Emissions:
Direct Emissions: Address emissions from sources owned or controlled by the company, such as fuel combustion in company-owned vehicles or manufacturing processes.
Reduction Strategies: Implement measures like upgrading energy-efficient equipment, switching to low-carbon fuels, and optimizing manufacturing processes.
2. Scope 2 Emissions:
Indirect Emissions: Focus on emissions from the consumption of purchased electricity, heat, and steam.
Energy Efficiency: Improve energy efficiency in buildings and operations to reduce electricity demand.
Renewable Energy: Purchase or generate renewable energy, such as installing solar panels or entering into power purchase agreements (PPAs) for wind or solar power.
3. Scope 3 Emissions:
Value Chain Emissions: Address all other indirect emissions, such as those from purchased goods and services, business travel, employee commuting, and the end-use of sold products.
Supplier Engagement: Work with suppliers to improve their environmental performance and reduce emissions. This can involve setting sustainability criteria for supplier selection.
Product Design: Design products for lower emissions during use and disposal, such as energy-efficient appliances or recyclable packaging.
Going Beyond Sustainable and Towards Regenerative
Regenerative practices aim to restore and enhance ecosystems rather than merely sustain them.
1. Regenerative Agriculture:
Soil Health: Practices like no-till farming, cover cropping, and rotational grazing improve soil health and increase carbon sequestration.
Biodiversity: Promote biodiversity through polyculture planting and habitat restoration, which can enhance ecosystem resilience and productivity.
2. Circular Economy:
Design for Reuse: Design products and systems to be reused, refurbished, remanufactured, and recycled. This reduces waste and conserves resources.
Material Cycles: Implement closed-loop systems where waste materials are continually cycled back into production processes.
3. Positive Impact Projects:
Environmental Restoration: Support projects that restore degraded ecosystems, such as reforestation, wetland restoration, and coral reef conservation.
Community Investment: Invest in projects that improve community well-being and resilience, such as sustainable urban development and renewable energy access in underserved areas.
4. Corporate Culture:
Sustainability Leadership: Cultivate leadership that prioritizes environmental and social restoration. Encourage innovative thinking and support initiatives that go beyond compliance.
Employee Engagement: Foster a culture of sustainability by involving employees in regenerative initiatives and recognizing their contributions to these efforts.
Optional: Tips on Building Employee Climate Action
Engaging employees in climate action is essential for embedding sustainability into the corporate culture.
1. Education and Awareness:
Training Programs: Provide training on sustainability practices and the company's climate goals. Use workshops, webinars, and e-learning platforms.
Awareness Campaigns: Run internal campaigns to raise awareness about climate change and the importance of sustainability. Use newsletters, posters, and social media.
2. Employee Involvement:
Green Teams: Establish employee-led green teams to drive sustainability initiatives and foster a sense of ownership.
Suggestion Programs: Encourage employees to contribute ideas for improving sustainability practices and recognize their contributions.
3. Incentives and Recognition:
Rewards Programs: Implement rewards programs to recognize employees who contribute significantly to sustainability efforts. This could include bonuses, public recognition, or other incentives.
Performance Metrics: Incorporate sustainability metrics into performance evaluations to align individual goals with corporate sustainability objectives.
4. Sustainable Practices:
Office Practices: Promote sustainable practices in the workplace, such as reducing paper use, implementing recycling programs, and encouraging energy conservation.
Sustainable Commutes: Provide incentives for using public transportation, carpooling, biking, or walking to work. Offer facilities like bike racks and showers.
SOMEONE CAN BENEFIT FROM IT
If you find this post helpful, we would be grateful if you could take a moment to share it with others who might benefit from it. Your kindness and support mean a lot to us.
DYNAMIC CONTENT
No one knows how deep a rabbit hole can go.
All our posts are regularly updated as soon as new information becomes available. We also keep note of the questions asked by our readers and add their answers to the article. So, stay curious and ask questions in the comments.
Last updated: 22 May 2024
ABOUT THE AUTHOR
Hey everyone, I'm Garvit Sahdev ๐. I'm on a mission to gain a deeper understanding of the world, and to develop solutions that can trigger significant global change.
My curiosities span various domains including food, business theories, material science, market size calculations, economics, politics, and sports, etc. ๐ง
Professionally, I have a diverse background spanning startups, consulting, policy development, market research, system building, ISO, colour physics, nanomaterial synthesis, textile chemistry, etc. ๐
Thanks for reading The Thoughtful Tangle! Subscribe to continue reading deeply researched stories about the interesting concepts that shape our world. โค๏ธ